Quantum Computing: Exploring the World of Quantum Computing
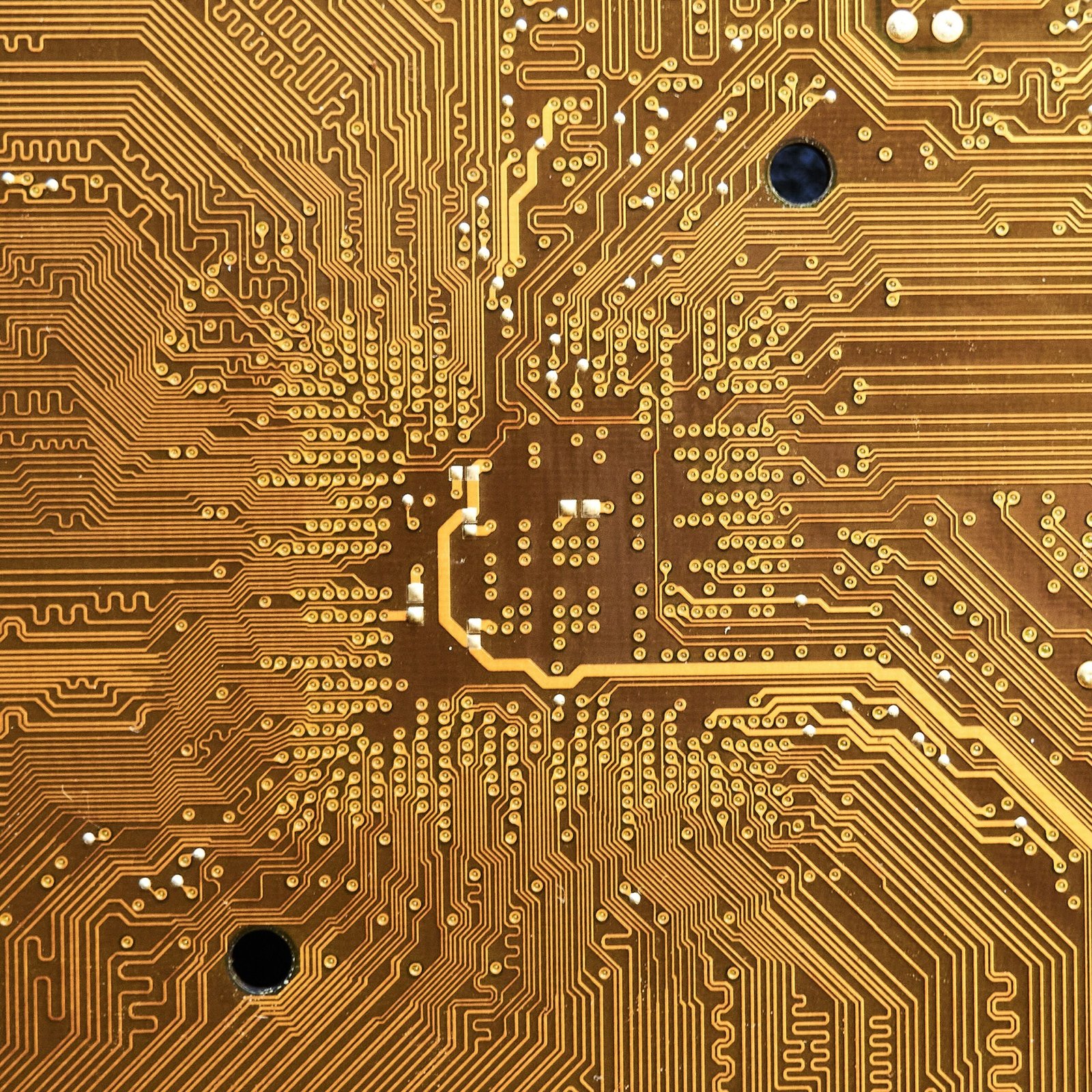
Introduction to Quantum Computing
Quantum computing represents a revolutionary advancement in the field of computation, diverging sharply from traditional classical computers. The fundamental unit of information in classical computing is the bit, which can exist in one of two states: 0 or 1. In contrast, quantum computing utilizes qubits, which can exist simultaneously in multiple states due to a property known as superposition. This allows quantum computers to process vast amounts of data simultaneously, increasing their computational power exponentially for certain tasks.
At the heart of quantum computing lies the principle of entanglement, another key concept that further distinguishes it from classical paradigms. When qubits become entangled, the state of one qubit becomes dependent on the state of another, regardless of the distance separating them. This interconnectedness enables quantum computers to tackle complex problems more efficiently than classical computers, which often operate sequentially.
The significance of quantum computing extends beyond mere speed; it introduces new computational approaches that could potentially solve problems that are currently intractable for classical machines. For example, quantum algorithms can efficiently factor large numbers or simulate molecular structures, offering profound implications for fields such as cryptography and materials science.
However, the journey towards practical quantum computing is not without challenges. Issues such as qubit decoherence and error rates must be addressed to construct reliable quantum systems. Research is ongoing, with experts exploring various quantum computing models, including gate-based quantum computing and quantum annealing. Each of these approaches aims to harness quantum mechanics’ unique properties to solve complex, real-world problems.
As we delve deeper into the intricacies of quantum computing, understanding these foundational concepts is crucial for appreciating the technology’s transformative potential and the impact it may have across various sectors.
The Science Behind Quantum Mechanics
Quantum mechanics represents a fundamental shift in our understanding of physics, delving into the behavior of matter and energy at the smallest scales. At the heart of quantum mechanics are the concepts of quantum states, which depict the information of quantum systems. Unlike classical systems, which have definite states, quantum states can exist in a superposition, wherein a particle can simultaneously assume multiple states until measured. This property is pivotal to quantum computing, as it allows for the execution of numerous calculations in parallel, a feat impossible for classical computers.
Another core concept is wave-particle duality, asserting that particles like electrons exhibit both wave-like and particle-like characteristics. This dual nature is crucial in quantum computing, permitting qubits— the fundamental units of quantum information—to function in a non-binary state. This capability enables qubits to store and process exponentially larger amounts of information, facilitating complex computations without the need for additional physical resources.
Moreover, Heisenberg’s uncertainty principle plays a significant role in understanding quantum mechanics. This principle states that certain pairs of physical properties, such as position and momentum, cannot be simultaneously measured with arbitrary precision. In the context of quantum computing, this means that while we can describe a qubit’s state, the exact values of its properties are inherently uncertain until a measurement occurs. This uncertainty does not hinder computation; rather, it enables the unique computational schemes that quantum computers leverage to solve problems.
Through these principles, quantum mechanics lays the groundwork for quantum computing, offering a glimpse into how these machines can perform calculations that transcend traditional binary systems. Understanding these foundational concepts is essential for exploring the capabilities and potential of quantum computers in various fields, from cryptography to complex simulations.
How Quantum Computers Work
Quantum computers operate on fundamentally different principles compared to classical computers. At the heart of quantum computing is the concept of qubits, which can exist in multiple states simultaneously due to the principle of superposition. Unlike classical bits, which can be either 0 or 1, qubits can represent both 0 and 1 at the same time, enabling quantum computers to process vast amounts of information with remarkable efficiency.
The architecture of a quantum computer typically involves several key components. Quantum gates serve as the building blocks for quantum circuits, analogous to classical logic gates. These gates manipulate qubits through transformations, which affect their probabilistic states. By applying various quantum gates, complex operations can be performed, facilitating tasks like encryption, optimization, and simulation of molecular structures.
In addition to quantum gates, the arrangement of these gates into quantum circuits is vital for developing algorithms that leverage quantum properties. Quantum circuits consist of a sequence of operations that are choreographed to solve specific problems, taking advantage of the unique behaviors of qubits. Algorithms like Shor’s and Grover’s illustrate the potential efficiencies that quantum processing can achieve, outpacing traditional computational methods for particular tasks.
Despite the promise of quantum technology, significant challenges remain in building functional quantum computers. Issues such as error rates, coherence times, and qubit connectivity must be addressed to develop stable and scalable systems. Additionally, the integration of quantum computing into practical applications requires advancements in both hardware and algorithm design, ensuring that the full potential of quantum computing can be realized. As research progresses, the ongoing development of quantum architectures and techniques will shape the future landscape of computing.
Applications of Quantum Computing
Quantum computing stands at the forefront of technological innovation, offering remarkable potential in various sectors. One of the most notable applications is in the field of cryptography. Traditional encryption methods rely on complex mathematical problems, like factoring large numbers, which can be efficiently solved by quantum algorithms such as Shor’s algorithm. This capability threatens current security protocols, prompting the development of quantum-resistant encryption methods to safeguard sensitive information.
Another promising application of quantum computing is in drug discovery. Quantum computers can perform simulations at unprecedented speeds, enabling researchers to analyze molecular interactions and predict the efficacy of potential drug candidates more accurately. For instance, pharmaceutical companies have begun to utilize quantum simulations to expedite the development of new medications, particularly in areas like cancer treatment and complex diseases, which often require extensive modeling.
Furthermore, quantum computing holds significant promise for solving optimization problems faced in various domains. Industries such as logistics, finance, and manufacturing encounter challenges that require efficient resource allocation and route optimization. Quantum algorithms can evaluate multiple solutions simultaneously, dramatically reducing the time it takes to find the optimal solution. Companies are already exploring how these advanced algorithms can revolutionize supply chain management and financial forecasting.
Lastly, the integration of quantum computing with artificial intelligence (AI) is an area garnering immense attention. Quantum machine learning algorithms can process vast amounts of data more rapidly than classical computers, enabling AI systems to uncover hidden patterns and enhance predictive analytics. This synergy could lead to breakthroughs in natural language processing, image recognition, and other AI applications, paving the way for more sophisticated and capable systems.
These applications illustrate the transformative power of quantum computing across diverse fields, revealing its potential to revolutionize industries, enhance security measures, and accelerate scientific discoveries.
Current State of Quantum Computing Technology
Quantum computing technology has garnered significant attention over the past few years, with numerous organizations striving to harness its potential for enhanced computation. Major players in the realm include technology giants such as IBM and Google, as well as a variety of startups and research institutions dedicated to advancing this cutting-edge field. These entities are motivated by the promise of quantum computers performing calculations that are currently infeasible for classical machines.
IBM, for instance, has made considerable strides with its Quantum Experience platform, which allows researchers and developers to access IBM’s quantum systems via the cloud. This initiative not only democratizes access to quantum computing but also fosters innovation by enabling collaboration among scientists and engineers globally. As of late 2023, IBM has introduced several iterations of quantum processors, each containing a growing number of qubits, thereby increasing computational power and potential applications.
Similarly, Google has displayed commendable progress in quantum technology, particularly with its Sycamore processor, which demonstrated quantum supremacy by performing a particular calculation faster than the world’s most advanced supercomputers could achieve. This milestone has intensified competition across the sector, spurring rival organizations to accelerate their research and development efforts.
In addition to these industry giants, other notable entities include startups like Rigetti Computing and IonQ, which have also made significant advancements in the field of quantum computing. Research institutions like MIT and Stanford University continue to contribute to the academic foundation underlying quantum advancements. The race in quantum technology not only reflects the ambition of these entities but also signifies a collaborative effort to explore new frontiers in computational capabilities.
In summary, the current landscape of quantum computing technology is marked by rapid advancements and increased competition among leading organizations and research centers. Collaborative efforts and groundbreaking innovations are paving the way toward the full realization of quantum computing’s potential and its transformative impacts across various sectors.
Challenges and Limitations of Quantum Computing
Quantum computing, despite its potential to revolutionize technology and industries, faces several significant hurdles that hinder its widespread adoption and practical application. One of the primary technical challenges is the high error rate associated with quantum calculations. Unlike classical bits, qubits are susceptible to noise and interference from their environment, leading to errors in quantum computations. Quantum error correction methods are being developed, but their implementation requires additional qubits, which complicates the overall architecture and maintenance of a quantum system.
Another critical limitation is qubit decoherence, which refers to the loss of quantum coherence in qubits due to environmental interactions. When qubits interact with external factors such as temperature fluctuations or electromagnetic radiation, they lose their quantum state and thus their computational capabilities. This decay occurs relatively quickly, making it difficult for quantum computers to perform complex operations that require a stable quantum state for an extended period. Researchers are actively working on methods to prolong coherence times, yet achieving this remains a formidable task.
Scalability is another pressing issue in quantum computing. Building practical quantum systems requires an increasing number of qubits, and each additional qubit introduces complexities in terms of control, error rates, and circuitry. The challenge lies in creating a scalable architecture that maintains a low error rate while allowing for efficient qubit interactions. Furthermore, there are theoretical limitations regarding computational complexity that may restrict the types of problems quantum computers can effectively solve.
Beyond technical challenges, there are public and regulatory concerns that accompany the development of quantum technology. These include questions about data privacy, security implications of quantum cryptography, and the ethical considerations surrounding advanced computational capabilities. As quantum computing technology progresses, addressing these challenges will be essential for realizing its potential in a responsible and sustainable manner.
The Future of Quantum Computing
As we look ahead to the next decade, the field of quantum computing is poised for transformative advancements that could revolutionize a multitude of sectors. One of the most anticipated developments is the expansion of quantum networks. These networks are expected to enable secure communication channels that leverage the principles of quantum mechanics, thereby providing a level of security unattainable by classical methods. With advancements in quantum key distribution and entanglement-based communication, organizations may soon be able to protect sensitive information with unparalleled efficacy.
Alongside the growth of quantum networks, breakthroughs in quantum algorithms are likely to redefine computational capabilities. Researchers are actively exploring novel algorithms that could solve complex problems—ranging from optimization tasks to simulations of molecular structures in pharmaceuticals—much faster than traditional approaches. For instance, algorithms like Shor’s and Grover’s could reach maturity, unlocking insights across industries such as cryptography and drug discovery. As companies begin to harness quantum computing resources more effectively, we may witness a significant acceleration in innovation and productivity.
Another pivotal aspect of the future of quantum computing is the notion of quantum supremacy. As technology progresses, we can expect systems to achieve this milestone at increasingly accessible levels, allowing more entities—from academic institutions to startups—to engage in quantum research. The implications of achieving quantum supremacy go beyond academic achievement; they herald the potential to solve real-world challenges that have thus far been intractable. This could ultimately inspire a new wave of technological advancements, paving the way for applications that can fundamentally alter our understanding of computation and information.
Thus, as the next decade unfolds, the advancements in quantum computing promise to reshape various sectors significantly. From enhanced security in quantum networks to the evolution of algorithms and the broader impact of quantum supremacy, the future seems not only bright but also filled with vast possibilities.
Quantum Computing vs Classical Computing
Quantum computing represents a radical shift from classical computing, fundamentally altering the way computational problems are approached. Classical computing relies on bits as the smallest unit of data, which can exist in one of two states: 0 or 1. In contrast, quantum computing uses quantum bits, or qubits, which can exist in multiple states simultaneously due to the principles of superposition and entanglement. This characteristic allows quantum computers to process a vast amount of information concurrently, offering potential solutions to complex problems that are intractable for classical computers.
One significant advantage of quantum computing is its ability to tackle specific problems faster than classical computers. For instance, quantum computers excel at factoring large numbers, which has implications for cryptography and security. They can also efficiently simulate quantum systems, making them invaluable for research across various scientific fields, including chemistry and materials science. These capabilities could lead to advancements in drug discovery and the development of new materials, consequently reshaping entire industries.
However, despite its advantages, quantum computing also faces challenges. Quantum systems are highly sensitive to environmental disturbances, leading to errors that require error correction mechanisms. Additionally, the technology is still in its infancy, with practical, widespread applications yet to be realized. In comparison, classical computing remains the backbone of current technology with its proven reliability and well-understood architecture.
In essence, while quantum computing presents the promise of revolutionizing computational capabilities, particularly for specific problems, classical computing continues to play a crucial role in everyday applications and processes. Both paradigms have their respective strengths and weaknesses, and their coexistence will likely foster advancements that enhance our technological landscape.
Getting Started with Quantum Computing
For those intrigued by quantum computing, the journey begins with a plethora of resources that cater to various learning styles and levels of expertise. Aspiring quantum enthusiasts can explore online courses that are specially designed for beginners to provide a solid foundation in the fundamentals of this advanced field. Platforms such as Coursera, edX, and Udacity offer courses in collaboration with leading universities and organizations, ensuring that learners receive up-to-date and relevant information about quantum theory, quantum mechanics, and algorithm design.
In addition to online courses, reading materials can significantly enrich one’s understanding of quantum computing. Several authors have produced excellent introductory texts that break down complex concepts into more manageable portions. Books such as “Quantum Computation and Quantum Information” by Michael A. Nielsen and Isaac L. Chuang are highly recommended for their comprehensive coverage of essential principles. Furthermore, for those interested in practical applications, “Quantum Computing for Computer Scientists” by Noson S. Yanofsky and Mirco A. Mannucci offers insights into how quantum computing intersects with traditional computing methodologies.
Research papers are another invaluable resource for those wanting to delve deeper into specific areas of quantum computing. Websites like arXiv.org host a wealth of preprints covering cutting-edge research and advancements in algorithms, quantum cryptography, and quantum hardware. Engaging with these papers can provide learners a more nuanced view of the current landscape and future directions of quantum computing.
Lastly, joining communities—be it online forums, social media groups, or local meetups—can facilitate meaningful interactions with fellow learners and experts. Platforms like Reddit, Stack Exchange, or dedicated Discord servers provide spaces for discussion and inquiry, allowing individuals to share knowledge and experience in this rapidly evolving field. By utilizing these resources, beginners can effectively embark on their quantum computing journey, equipping themselves with the tools necessary to navigate this complex domain.