Advancing Nanoelectronics: Overcoming the Diffraction Limit
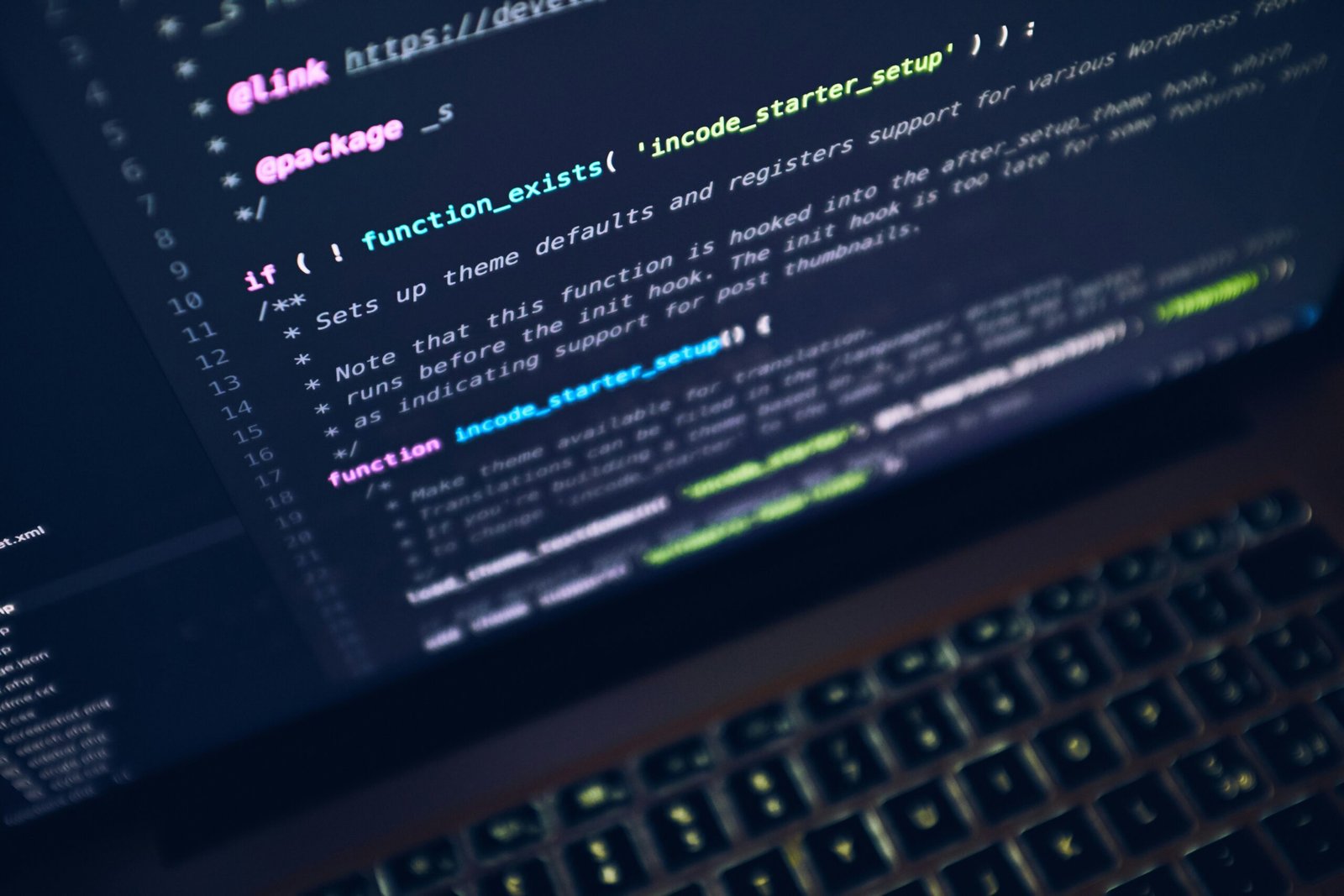
Introduction to Nanoelectronics
Nanoelectronics is a branch of electronics that leverages the unique properties of materials at the nanoscale to develop devices with enhanced functionality and performance. At its core, nanoelectronics focuses on the manipulation of matter at dimensions typically ranging from one to a few hundred nanometers. This scale is critical as it allows for the exploration of quantum mechanical effects, which govern the behavior of materials when they are reduced to such small dimensions. Unlike traditional electronics, which relies primarily on bulk properties, nanoelectronics exploits the distinctive electronic, optical, and magnetic properties of nanostructures, paving the way for innovations that were previously unattainable.
The significance of nanoelectronics extends far beyond mere miniaturization of electronic components. By facilitating the creation of smaller and more efficient devices, nanoelectronics has the potential to revolutionize multiple industries. For instance, in computing, nanoelectronic components can lead to faster data processing speeds and reduced power consumption, providing a solution to the challenges posed by the growing demand for efficient and high-performance computing systems. Similarly, in communications, the ability to manipulate signals at the nanoscale promises enhancements in bandwidth and data transfer rates, enabling more robust and sophisticated networks.
Moreover, nanoelectronics plays a vital role in data storage technologies. As the volume of data generated globally continues to rise exponentially, traditional storage solutions face limitations in capacity and speed. Nanoelectronic devices can bridge this gap by enabling higher density storage solutions and faster retrieval times, thus addressing the critical demands of industries reliant on big data analytics. In conclusion, the advancement of nanoelectronics is poised to transform modern technology across various fields, reinforcing its essential role in future developments of electronic devices.
Understanding the Diffraction Limit
The diffraction limit is a fundamental principle in optics that defines the smallest resolvable feature size in an imaging system. It arises from the wave nature of light, particularly its tendency to spread or diffract upon encountering an obstacle or aperture. The essential relationship governing the diffraction limit is expressed by the equation: resolution ∝ λ / D, where λ represents the wavelength of light and D denotes the aperture diameter. This equation highlights that both the wavelength of light used and the size of the aperture play critical roles in dictating the achievable spatial resolution.
The implications of the diffraction limit are profound in the field of nanoelectronics, especially as devices continue to shrink to the nanoscale. Given that many optical systems utilize visible light, which has a wavelength range of approximately 400 to 700 nanometers, the capacity for resolving features smaller than this wavelength becomes increasingly difficult. Consequently, conventional optical microscopy encounters significant challenges when attempting to visualize structures at atomic or molecular scales. Structures that lie beneath the diffraction limit remain obscured, complicating efforts for manipulation and analysis at the nanoscale.
Real-world applications demonstrate the repercussions of this limitation. For instance, in semiconductor manufacturing, the ability to observe and engineer nanoscale components is crucial for the development of advanced materials and devices. The constraints imposed by the diffraction limit necessitate the exploration of alternative techniques, such as electron microscopy, which utilizes electron wavelengths much shorter than visible light. This shift enables researchers to bypass the traditional optical challenges and enhance the resolution of their observations. However, the transition from optical methods to electron-based systems introduces new complexities and trades off certain advantages, underlining the intricate nature of addressing the diffraction limit in modern technologies.
The Importance of Overcoming the Diffraction Limit
In the field of nanoelectronics, the diffraction limit poses a significant challenge to the development and operational efficiency of electronic components. The diffraction limit refers to the inability of traditional optical imaging techniques to resolve objects smaller than a certain wavelength of light. As devices shrink to the nanometer scale, this limitation hampers the capacity to accurately manipulate and integrate components, thus hindering the progression of technology. Overcoming the diffraction limit is therefore crucial for advancing nanoelectronics.
Improving chip operation is one of the primary benefits of surpassing the diffraction limit. As electronic components become smaller, the performance of integrated circuits can be compromised due to increased resistance, capacitance, and other parasitic effects. By employing techniques that circumvent the diffraction limit, engineers can design chips that are not only more compact but also demonstrate enhanced performance capabilities. The ability to visualize and manipulate structures at the nanoscale would facilitate the creation of more efficient pathways for electrons, ultimately resulting in faster processing speeds and reduced energy consumption.
Moreover, overcoming the diffraction limit also has profound implications for data storage capabilities. Current storage technologies often struggle with density due to the constraints imposed by optical imaging. If we can develop methods that allow for finer resolution of data writing and reading processes, we can achieve unprecedented data density in storage devices. This advancement would enable the storage of significant amounts of information in smaller physical spaces. Furthermore, advancements in nanoelectronics will allow for the development of new materials and architectures that can support faster and more efficient devices, which is vital for the burgeoning industry of smart systems and the Internet of Things.
The impact of overcoming the diffraction limit extends beyond immediate benefits for current technologies. It lays the groundwork for future innovations, opening doors to entirely new paradigms in electronic device design and functionality. As researchers continue to explore and implement solutions, the advancement of nanoelectronics stands to revolutionize not just computing, but a wide array of sectors from healthcare to communications.
Current Techniques to Surpass the Diffraction Limit
The diffraction limit has traditionally posed significant challenges in the field of nanoelectronics, restricting the resolution and performance of nanostructures. However, researchers have continuously sought innovative methods to overcome this limitation. Among the foremost techniques is near-field optics, which utilizes a unique approach to manipulate light beyond the conventional diffraction limit. By employing localized electromagnetic fields, near-field optics enables the visualization and manipulation of materials at an unprecedented scale. This technique is particularly beneficial in characterizing nanoscale devices that form the backbone of nanoelectronics.
Another pivotal method is superresolution microscopy, which encompasses various techniques such as Stimulated Emission Depletion (STED) microscopy and Single-Molecule Localization Microscopy (SMLM). These advanced imaging methods permit imaging at resolutions significantly finer than what traditional light microscopy can achieve. For instance, STED microscopy applies a doughnut-shaped laser beam to deactivate fluorescence in an area surrounding a focal point, allowing the observation of structures smaller than the diffraction limit. SMLM, on the other hand, involves the precise localization of individual fluorophores, providing high-resolution images crucial for the analysis of nano-scale electronic components.
Additionally, techniques such as electron microscopy have greatly advanced, enabling imaging of materials at atomic resolutions. The ability to visualize nanoelectronic devices at such high fidelity aids in understanding and optimizing the electronic properties of materials, leading to enhanced performance. Furthermore, advanced lithography techniques, including extreme ultraviolet (EUV) lithography, play a vital role in the fabrication of nanoscale devices. By harnessing light of shorter wavelengths, these methods enable the creation of patterns with dimensions smaller than the diffraction limit, paving the way for new developments in nanoelectronics.
These various approaches demonstrate the ongoing efforts within the scientific community to push the boundaries of resolution in nanoelectronics. By integrating these techniques, researchers are gradually surmounting the obstacles presented by the diffraction limit, fostering significant advancements in the design and functionality of nanostructured devices.
Challenges in Nanoelectronics Research and Development
The realm of nanoelectronics, while promising groundbreaking advancements, is beset by a myriad of challenges that complicate both research and development efforts. One of the foremost hurdles lies in the high costs of materials. The substances required for constructing nanoscale devices are often expensive, and acquiring them in sufficient quantities for experimental and production purposes can strain research budgets significantly. In addition to the financial implications, the purity and quality of these materials are crucial, as even minor impurities can lead to major defects in performance.
Fabrication techniques represent another significant challenge in the nanoelectronics sector. Traditional methods of semiconductor manufacturing, such as photolithography, struggle to meet the requirements of processes at the nanoscale due to the diffraction limit of light. As researchers delve deeper into the nanoscale realm, alternative techniques, such as electron-beam lithography, are being explored, yet these methods come with their own set of limitations, including prolonged processing times and increased complexity. The inherent difficulty of achieving desired patterns and features at such a diminished scale impacts throughput and cost-effectiveness in production.
Moreover, the necessity for precision during fabrication cannot be overstated. At the nanoscale, even the most minimal deviations can considerably affect the electrical and mechanical properties of the resulting devices. This demand for exactitude extends beyond mere production; it also encompasses testing and characterization techniques to ensure that these tiny components perform reliably across varying conditions. The convergence of scientific understanding and engineering prowess is essential in overcoming these intricacies, indicating that the journey toward commercializing nanoelectronics is a multifaceted one that requires continuous innovation and collaboration among experts in these fields.
Future Prospects for Nanoelectronics
As researchers continue to explore the frontiers of nanoelectronics, the potential developments in this field are poised to revolutionize various industries. Overcoming the diffraction limit represents a significant milestone that could pave the way for enhanced performance and capabilities in electronic devices. Emerging trends such as the integration of materials with unique properties, like graphene and other two-dimensional materials, are expected to enhance the efficiency of components used in nanoelectronic applications. These advancements can lead to smaller, faster, and more energy-efficient devices, bringing forth a new era of electronics.
In computing, the implications of overcoming the diffraction limit could lead to the development of quantum computing systems with unprecedented processing power. As traditional silicon-based transistors reach their physical limits, innovations in nanoelectronics may introduce new paradigms that leverage quantum mechanics for data processing. This transition could profoundly impact industries that rely on computational speed and data analysis, such as artificial intelligence and machine learning.
Telecommunications is another sector that stands to benefit significantly from advancements in nanoelectronics. Enhanced signal processing capabilities combined with the miniaturization of components could result in improved data transmission rates. This improvement is especially relevant in the context of 5G and future wireless technologies, which require high bandwidth and low latency. Nanoelectronic devices designed to operate at higher frequencies could support the increasing demands for connectivity and facilitate the growth of the Internet of Things (IoT).
Moreover, the biomedical field may experience transformative changes due to the capabilities provided by nanoelectronics. Innovations in sensors at the nanoscale can lead to more precise diagnostic tools, enabling early detection of diseases. The integration of nanoelectronic systems in medical devices could also enhance monitoring and treatment options for patients, increasing the effectiveness of interventions.
As we look ahead, the convergence of these advancements suggests a promising future for nanoelectronics, placing it at the forefront of technological evolution across diverse sectors.
Case Studies: Successful Implementation of Advanced Nanoelectronics
The realm of advanced nanoelectronics has witnessed significant transformations, particularly in applications that aim to surmount the diffraction limit. Real-life case studies provide insight into how these innovations are effectively implemented across multiple sectors, culminating in substantial improvements and groundbreaking developments.
One notable example is the use of nanoelectronic circuits in the field of telecommunications. Researchers at a leading tech institute successfully developed a nanoscale optical switch that operates at unprecedented speeds. This technology utilizes photonic crystal structures that harness electromagnetic waves beyond traditional optical limits, facilitating faster data transmission rates. The success of this implementation demonstrates that overcoming the diffraction limit can pave the way for more efficient communication networks, which have become indispensable in today’s data-driven environment.
In the automotive industry, advanced nanoelectronics have transformed vehicular technology. A prominent automotive manufacturer integrated nanoelectronic sensors into their vehicles to enhance safety and performance. These sensors, capable of detecting minute changes in environmental conditions, utilize sophisticated materials that operate effectively at nanometer scales. The implementation of this technology not only improved the reliability of the vehicles but also contributed to the development of autonomous driving systems. This case study illustrates how nanoelectronics is revolutionizing industrial applications and driving advancements in smart technology.
Another significant case study can be found in medical diagnostics. A pioneering biotechnology company has leveraged advanced nanoelectronic devices to enhance medical imaging techniques. By employing nanoscale imaging agents, they were able to achieve superior resolution and specificity in detecting cellular anomalies. This advancement not only showcases the feasibility of overcoming the diffraction limit but also signifies the potential for significant improvements in early disease detection and patient care.
These examples underscore the transformative impact of advanced nanoelectronics. As industries continue to adopt this technology, the implications for efficiency, safety, and accuracy are likely to grow exponentially, marking a new era in technological innovation.
Collaboration and Innovation in Nanoelectronics
The field of nanoelectronics stands at the forefront of technological advancement, grappling with the inherent challenges posed by the diffraction limit. Overcoming these challenges necessitates robust collaboration among various stakeholders, including academia, industry, and research institutions. Each of these entities plays a unique role in fostering innovation and addressing the complexities inherent in nanoelectronics.
Academic institutions serve as hotbeds of research and discovery, often pioneering basic research that informs the development of new nanoelectronic materials and devices. Collaborating with industry allows researchers to gain practical insights into the commercial applicability of their work, ensuring that their innovations can transition from the lab to the marketplace. Conversely, industry partners benefit from the latest findings from academia, integrating cutting-edge research into their product development processes. Such partnerships have proven essential in scaling innovative solutions that can effectively address the limits imposed by current technology.
The integration of interdisciplinary approaches is equally crucial. Nanoelectronics, by its very nature, intersects various fields such as materials science, physics, and engineering. Thus, collaboration that transcends traditional disciplinary boundaries can lead to more holistic and effective solutions. Interdisciplinary teams have the potential to bring diverse perspectives together, catalyzing the development of novel techniques and processes that push the boundaries of nanoelectronics. From joint research projects to shared facilities, the collaborative spirit fosters an environment ripe for innovation.
In addition to academia and industry, research institutions also play an instrumental role. They often facilitate collaborative networks that connect various stakeholders, providing frameworks for dialogue, resource sharing, and coordinated research efforts. By bringing together expertise from different sectors, these institutions can enhance the potential for breakthroughs in nanoelectronics, ultimately leading to advancements that may have seemed unattainable.
Conclusion: The Path Forward for Nanoelectronics
The rapid evolution of nanoelectronics has opened up new avenues for technological advancement, particularly by addressing the inherent challenges posed by the diffraction limit. This limit, primarily related to the constraints of optical resolution, has historically hindered the development of smaller and more efficient electronic components. However, the innovations in advanced imaging techniques and materials science are paving the way for breakthroughs that can significantly enhance the performance and capabilities of nanoelectronics.
As the demand for smaller, faster, and more efficient electronic devices increases, overcoming the diffraction limit is crucial. Researchers are now exploring novel methods, including the use of alternative fabrication techniques and advanced materials that display unique electrical properties at the nanoscale. These approaches not only aim to push the boundaries of physical dimensions but also seek to improve energy efficiency and overall device performance.
Furthermore, the collaboration between academia, industry, and research institutions will play a key role in fostering innovation in this field. By promoting interdisciplinary research initiatives, we can accelerate the discovery of new technologies that challenge conventional limitations. The integration of artificial intelligence and advanced simulations will also contribute to more efficient design processes and material discovery, propelling the field of nanoelectronics toward unprecedented advancements.
In conclusion, the future of nanoelectronics lies in our ability to surmount the challenges imposed by the diffraction limit. Continuous research and advancements in technology are imperative for achieving significant developments that will ultimately transform electronic devices as we know them. The ongoing commitment to innovation will not only enrich current technologies but will also lay a robust foundation for future discoveries in the realm of nanoelectronics, shaping a more connected and efficient world.