Harnessing Ferroelectric Domain Walls for Brain-Inspired Neuromorphic and In-Memory Computing
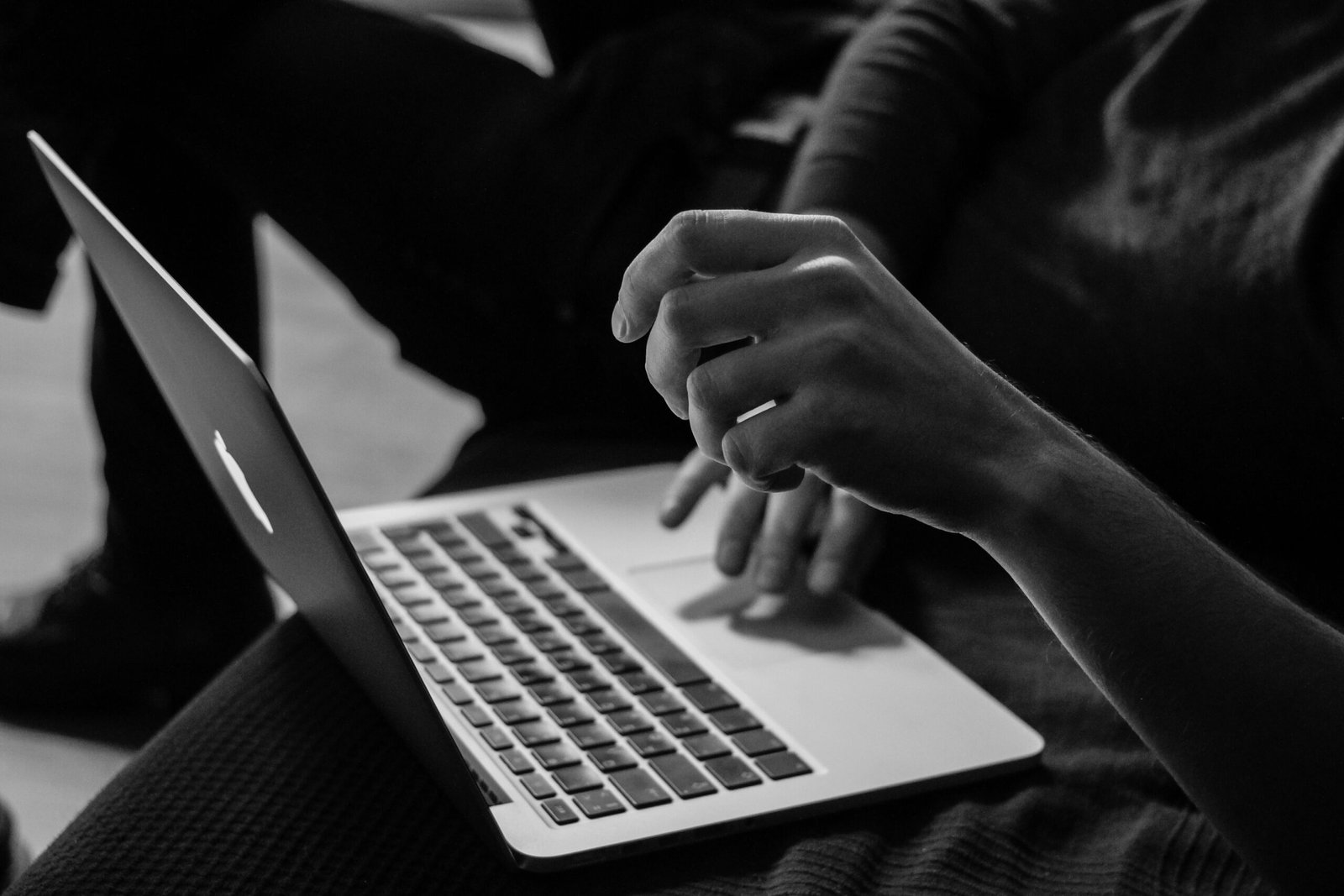
Introduction to Neuromorphic and In-Memory Computing
Neuromorphic computing represents a paradigm that seeks to emulate the neural structures and processing mechanisms of the human brain. It is an innovative approach to computing designed to enhance the efficiency and performance of computational processes, particularly in fields such as artificial intelligence and machine learning. By mimicking the architecture of biological nervous systems, neuromorphic systems aim to replicate the way humans process information, enabling machines to learn, adapt, and perform tasks with greater autonomy. The key components of neuromorphic computing include neurons, synapses, and the dynamic connections between them, which work together to replicate cognitive functions.
Complementing neuromorphic computing is in-memory computing, a technology designed to optimize data processing by significantly reducing latency. Traditional computing architectures often face bottlenecks as data must frequently travel between memory and processing units. In-memory computing addresses this challenge by bringing computation closer to data storage, allowing operations to be performed directly within the memory. This minimizes data transfer distances, leading to enhanced processing efficiency, particularly for large datasets commonly leveraged in machine learning applications.
The integration of neuromorphic and in-memory computing technologies signifies a major advancement in modern computing paradigms. With the rapid growth of applications in artificial intelligence, these complementary technologies promise to facilitate more efficient neural networks capable of learning and adapting in real-time. This integration not only enhances computational efficiency but also addresses the increasing demands for energy-efficient processing solutions. As researchers continue to explore the capabilities of ferroelectric domain walls in this context, the potential for revolutionary advancements in neuromorphic and in-memory computing remains significant, paving the way for smarter, more capable computational systems.
The Role of Ferroelectric Materials
Ferroelectric materials play a crucial role in advancing neuromorphic and in-memory computing systems due to their unique electrical properties. One of the defining characteristics of these materials is spontaneous polarization, which refers to the polarization that occurs without an external electric field. This property enables ferroelectric materials to maintain a stable electric dipole moment, thereby allowing for data storage capabilities analogous to biological neurons. Additionally, the reversible electric polarization that ferroelectrics exhibit facilitates efficient read and write operations, making them suitable for memory applications.
The ability of ferroelectric materials to switch their polarization states under the influence of external electric fields is particularly significant. This switching behavior allows for the embedding of binary information within the material, which can be read back with minimal energy loss. As a result, ferroelectric materials have emerged as potential candidates for creating non-volatile memory devices, providing an alternative to traditional semiconductor technologies. Their compatibility with complementary metal-oxide-semiconductor (CMOS) processes further establishes their viability in modern electronics.
In the realm of neuromorphic computing, ferroelectric materials emulate neuronal behavior by leveraging their polarization properties to simulate synaptic connections and firing patterns. Such behavior lends itself well to the development of artificial neural networks that can learn and adapt dynamically to input stimuli. By mimicking the synaptic weight changes seen in biological systems, ferroelectric devices can process and store information in a manner that is not only efficient but also fundamentally analogous to the operation of the human brain. This capability opens up new avenues for developing advanced computing systems that require lower power consumption and faster operational speeds across various applications, including artificial intelligence and machine learning.
Understanding Ferroelectric Domain Walls
Ferroelectric domain walls represent a crucial aspect of ferroelectric materials, which are known for their unique ability to exhibit spontaneous electric polarization that can be reversed by the application of an external electric field. A ferroelectric material consists of regions called domains, each possessing a uniform polarization direction. The boundaries between these differently polarized regions are referred to as domain walls. These walls are not just passive structures; they play an integral role in influencing the overall electronic properties of the material.
The formation of ferroelectric domain walls occurs during the phase transitions of ferroelectric materials, typically when they are subjected to temperature changes or external electric fields. Upon cooling below a certain temperature, known as the Curie temperature, the material undergoes a change that allows it to develop spontaneous polarization in one direction, leading to the establishment of distinct polarization domains. When external perturbations or changes in environmental conditions are introduced, these domain walls can shift or be reconfigured, effectively modifying the polarization landscape of the material.
The significant property of ferroelectric domain walls is that they can be manipulated through external electric fields, allowing for precise control over the material’s polarization states. This technique opens up new avenues for data storage and processing applications. By positioning domain walls strategically, it becomes feasible to create stable and reconfigurable data states, which are essential for memory and logic operations in neuromorphic computing systems. Additionally, the ability to control these walls enhances the potential for efficient in-memory computing architectures, whereby data is processed directly where it is stored, minimizing latency and improving energy efficiency.
Innovative Techniques for Manipulating Domain Walls
Recent advancements in the field of ferroelectric materials have paved the way for innovative techniques aimed at manipulating the shape and position of single ferroelectric domain walls. These domain walls, which separate regions of different polarization within a ferroelectric material, are critical in the development of neuromorphic and in-memory computing systems. Researchers are employing various methods, including the application of electric fields, temperature modulation, and mechanical stress, to achieve precise control over these domain walls.
Applying electric fields represents one of the most energetic methods for domain wall manipulation. By utilizing localized electric fields, researchers can induce transitions in the polarization states of the ferroelectric material, effectively causing domain walls to shift. This technique allows for fine-tuning of the position of these walls, which plays a significant role in their use for encoders and data retrieval systems. Furthermore, electric-field-induced domain wall movement can be harnessed for developing memories that exhibit low power consumption, a key feature for neuromorphic computing applications.
Temperature modulation also serves as an effective approach for manipulating ferroelectric domain walls. Variations in temperature can alter the properties of the ferroelectric material itself, thereby impacting the behavior of domain walls. By heating or cooling specific regions, researchers have demonstrated the ability to control the width and shape of these barriers, enabling the creation of domain wall-based devices with unique functionalities. This adaptability is particularly advantageous, as it allows for the scaling of devices and the potential for multi-state memory systems.
Additionally, the application of mechanical stress is emerging as another promising technique. Stress-induced manipulation of domain walls facilitates not only their movement but also the configuration of ferroelectric structures. The ability to combine these different techniques expands the toolbox available for researchers, unlocking new pathways for efficient data encoding and retrieval. As these techniques continue to develop, they will contribute significantly to the evolution of neuromorphic computing technologies.
Applications of Integrated Ferroelectric Devices
The integration of ferroelectric devices that exploit domain walls has emerged as a transformative force in various advanced computing fields, particularly neuromorphic and in-memory computing. These devices offer a unique approach to emulate the functionality of the human brain, enabling the design of artificial intelligence systems that can process and learn from data more naturally and efficiently. By leveraging their inherent properties, integrated ferroelectric devices facilitate rapid and low-power computation, making them ideal for a multitude of applications.
One of the most notable applications of integrated ferroelectric devices is in the realm of robotics. Autonomous robots rely heavily on efficient data processing to carry out tasks such as navigation, object recognition, and interaction with their environment. The reduced power consumption and high-speed operation of ferroelectric devices can significantly extend the operational lifespan of robotic systems, allowing them to achieve complex tasks without the limitation of energy constraints. Moreover, the ability of these devices to learn and adapt in real-time mimics human cognitive capabilities, enabling robots to operate more intuitively.
In addition to robotics, integrated ferroelectric devices play a vital role in data analysis, particularly in big data environments where traditional computing architectures may falter. Their memory efficiency allows for faster access and processing of large data sets, thereby accelerating analytical tasks across various industries such as finance, healthcare, and marketing. The incorporation of neuromorphic computing principles through ferroelectric technology can lead to breakthroughs in real-time data analysis and decision-making, promoting intelligent systems that evolve as they learn.
Consequently, these applications illustrate the potential of integrated ferroelectric devices in enhancing computational efficiency and speed across diverse fields. Their contributions pave the way for innovative solutions in artificial intelligence and beyond, highlighting their significance in the future of technology.
Advantages of Using Ferroelectric Domain Walls
Ferroelectric domain walls present a transformative approach in the realm of computing systems, offering several compelling advantages that position them favorably against traditional silicon-based technologies. One of the most significant benefits is their potential for lower power consumption. In an era where energy efficiency is paramount, the ability of ferroelectric materials to retain polarization states with minimal energy input is highly advantageous. This characteristic allows for significant reductions in the overall power required for data processing and storage, making them an efficient alternative for future computing needs.
In addition to enhancing energy efficiency, ferroelectric domain walls exhibit increased processing speed. Traditional transistor-based systems often face limitations due to the inherent capacitance and resistance that slow down data transfer rates. In contrast, the movement of domain walls within ferroelectric materials can occur at much higher speeds, enabling rapid switching capabilities that facilitate faster computation rates. This elevated speed can be crucial in applications that demand high-performance processing, such as real-time data analysis and machine learning.
Moreover, the flexibility in data storage offered by ferroelectric domain walls is another notable advantage. Unlike conventional binary systems that rely on discrete values for information storage, ferroelectric materials can leverage multiple polarization states. This characteristic not only allows for denser storage of data but also enhances the richness of information encoding. Such adaptability is particularly beneficial for neuromorphic computing systems, which aim to mimic the human brain’s efficiency and flexibility in data handling.
In conclusion, the integration of ferroelectric domain walls into computing architectures can significantly improve the performance metrics regarding power efficiency, processing speed, and storage flexibility. As research continues to explore these advantages, it becomes increasingly evident that ferroelectric materials may play a pivotal role in the future of computing technology, providing viable alternatives to traditional silicon-based systems.
Challenges and Limitations
The research and application of ferroelectric domain walls in neuromorphic and in-memory computing present several challenges and limitations that must be addressed to facilitate their practical adoption. One of the primary concerns is material degradation. Ferroelectric materials, while promising, can suffer from fatigue and instability over time when subjected to repeated electric field cycles. This degradation may affect device reliability and longevity, posing significant hurdles for their integration into long-term operational systems.
Another challenge is the integration of ferroelectric domain walls with existing semiconductor technologies. Current manufacturing processes for semiconductor devices are well-established, offering optimized performance characteristics maximized for traditional architectures. The incorporation of ferroelectric materials necessitates a fundamental reevaluation of fabrication techniques, which could lead to increased complexity and costs in semiconductor fabrication. Compatibility with existing materials and processes is crucial to maintain yield and functionality within integrated circuits.
Scalability represents a further limitation. As the demand for more powerful and efficient computing systems grows, the ability to produce ferroelectric devices at scale is essential. Current research primarily focuses on nanoscale applications, but translating these findings into commercially viable products requires significant advances in fabrication techniques and strategies. Moreover, achieving the desired performance characteristics in larger systems without compromising efficiency is a key obstacle that researchers are striving to overcome.
To tackle these challenges, ongoing investigations are exploring novel material compositions, advanced fabrication methods, and strategies for hybrid integration. Solutions such as developing improved compositions that exhibit enhanced cycling stability and exploring layered structures may provide pathways for overcoming some of these limitations. Continued interdisciplinary collaboration among physicists, materials scientists, and engineers will be vital in addressing these issues and advancing the field of neuromorphic computing.
Future Directions in Research
The exploration of ferroelectric domain walls is on the cusp of significant advancements, particularly in the context of neuromorphic and in-memory computing. As the field evolves, researchers are focusing on identifying innovative materials that can effectively support the unique properties of ferroelectric domain walls. These materials not only play a pivotal role in enhancing computational capabilities but are also integral to the advancement of energy-efficient computing systems. Future research is expected to delve into the development of novel ferroelectric materials that exhibit superior switching characteristics, which could lead to breakthroughs in speed and performance.
Another promising avenue for investigation lies in interdisciplinary collaboration, where physicists, materials scientists, and computer engineers work together to fully unlock the potential of ferroelectric technology. By merging insights from various fields, it may be possible to create hybrid systems that leverage the advantages of ferroelectric domain walls alongside complementary technologies such as memristors or quantum computing elements. This integration could facilitate more sophisticated neural architectures akin to the human brain, enabling enhanced data processing and more efficient pattern recognition systems.
Moreover, the advent of machine learning and artificial intelligence presents a tremendous opportunity to harness ferroelectric domain walls for novel applications. Researchers might focus on embedding these domain walls within neural networks, allowing for a more adaptive and self-learning computational framework. The ability to manipulate domain walls in real-time adds another layer of complexity, potentially resulting in systems capable of rapid learning and adjustment, echoing the inherent flexibility of biological neural networks.
As the landscape of computing technology continues to expand, it is imperative to keep exploring these future research directions. The continued evolution of ferroelectric domain walls will be crucial for realizing innovative computing paradigms that resonate with the demands of modern society.
Conclusion
The exploration of ferroelectric domain walls has opened new avenues in the fields of neuromorphic and in-memory computing. These domain walls, which are unique structural features present in ferroelectric materials, exhibit a remarkable ability to manipulate charge and polarization at the nanoscale. This capability holds significant promise for the development of brain-inspired computing architectures that emulate the fundamental functionalities of biological neural networks. By leveraging the unique properties of ferroelectric materials, researchers are beginning to forge pathways toward more efficient, adaptable, and powerful computational systems.
Throughout this discussion, we have highlighted the potential advantages provided by ferroelectric domain walls, such as low energy consumption, rapid switching speeds, and compatibility with current semiconductor technologies. These characteristics suggest that employing ferroelectric materials could lead to significant advancements in the efficiency and performance of computing systems, potentially enabling them to perform complex tasks with speed and efficiency that rival human cognitive functions. Moreover, by integrating these technologies with existing in-memory computing paradigms, we can expect improvements in data processing capabilities, paving the way for innovations in machine learning and artificial intelligence.
As ongoing research delves deeper into the mechanisms and applications of ferroelectric domain walls, it is crucial to invest in innovation and interdisciplinary collaboration. By combining expertise from materials science, electrical engineering, and computational theory, it becomes possible to unlock the full potential of these unique materials. The pursuit of harnessing ferroelectric domain walls is not just an academic endeavor; it represents a fundamental shift in our approach to computing that could herald a new era of smart technologies, mirroring the computational proficiencies of the human brain.